Dysregulated CARD11 signaling in the development of diffuse large B cell lymphoma
Abstract
Introduction

CARD11 signaling to NF-κB
mTOR and Akt-FOXO1 signaling
JNK signaling
Key role of E3 ligases and deubiquitinases
Bridging BCR and TLR signaling
Therapies targeting CARD11 signaling
Conclusion
REFERENCES
Information & Authors
Information
Published In
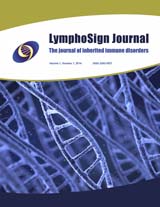
History
Copyright
Authors
Metrics & Citations
Metrics
Other Metrics
Citations
Cite As
Export Citations
If you have the appropriate software installed, you can download article citation data to the citation manager of your choice. Simply select your manager software from the list below and click Download.
There are no citations for this item
View Options
View options
Login options
Check if you access through your login credentials or your institution to get full access on this article.