Host–microbe interactions in the gut: lessons learned from models of inflammatory bowel diseases
Abstract
Introduction
Microbial involvement in health and disease (focus on inflammatory bowel diseases)
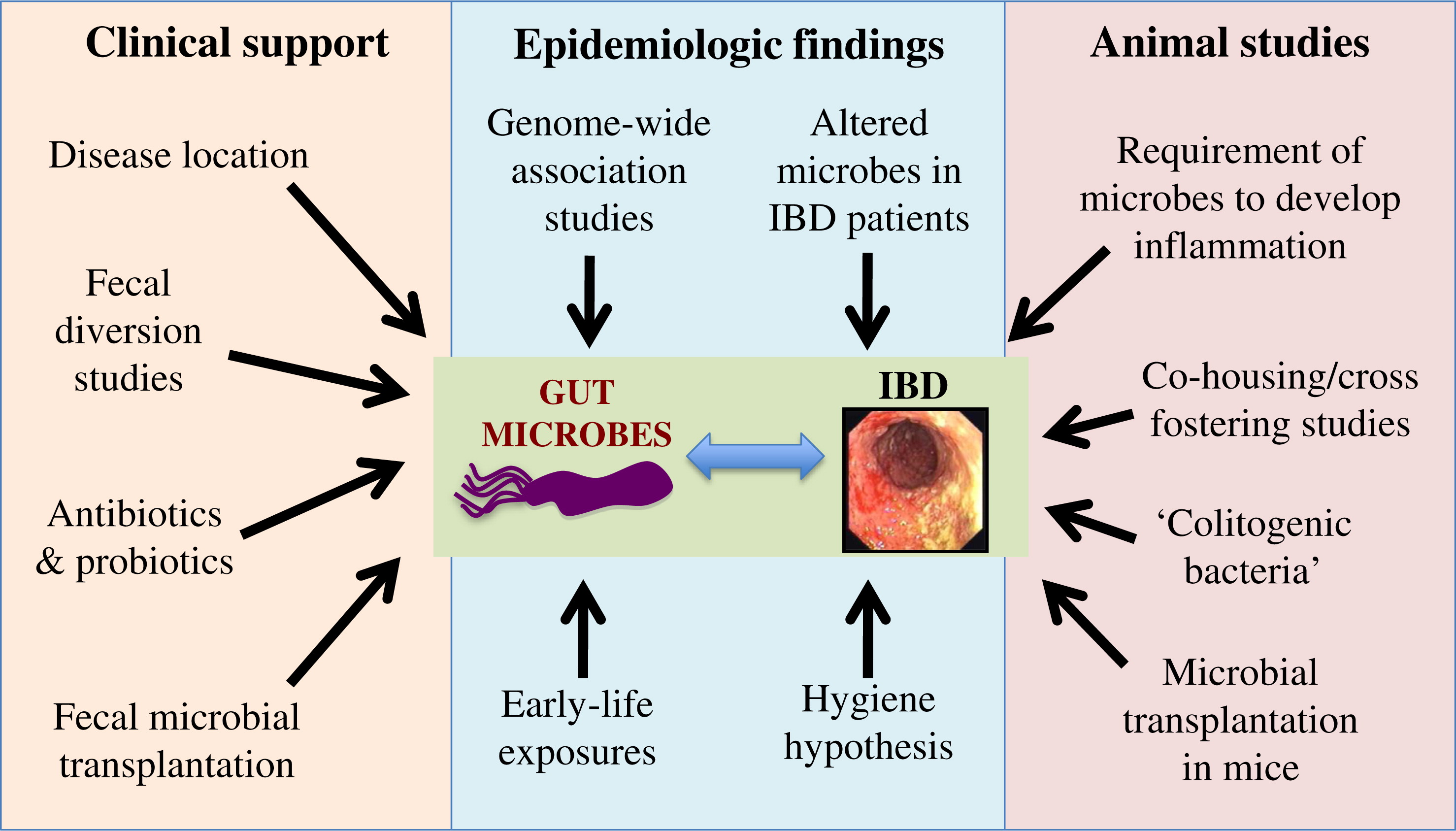
Microbes and IBD pathogenesis
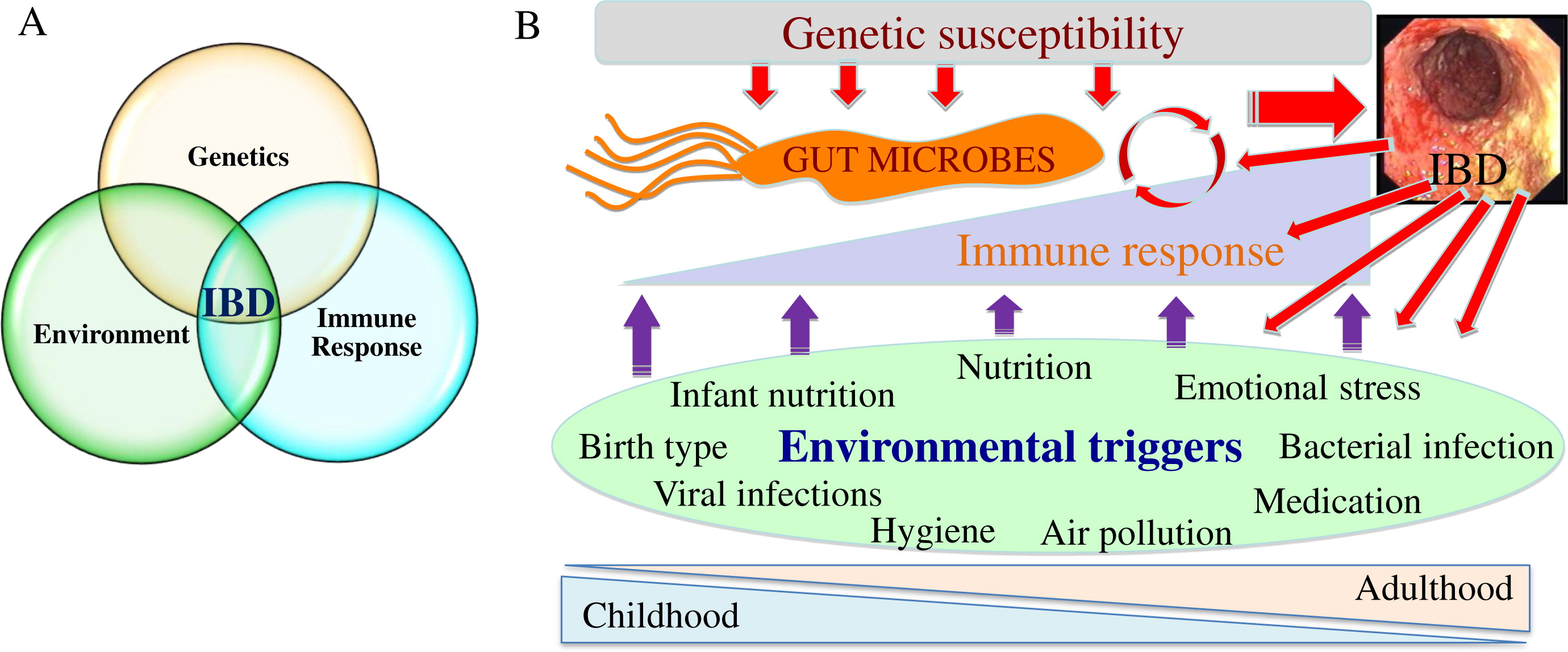
Innate immunity and host defence: bridging between the host and microbes
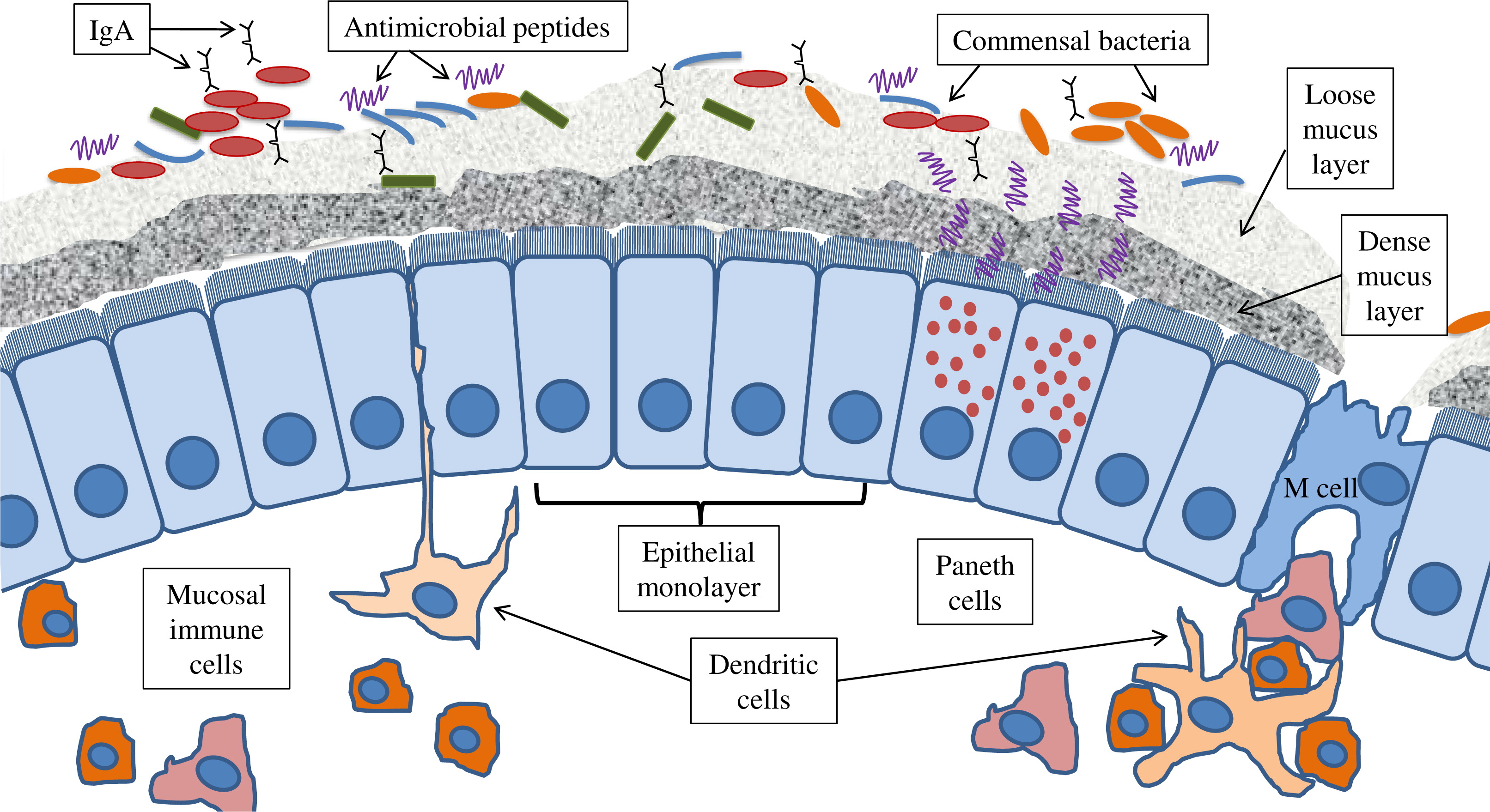
Microbial composition and function in IBD patients
Advancements in research tools used to study microbes and their effects
Using host–microbe research to advance patient care
Microbial composition-guided therapies
Manipulating host immune responses using microbes and nutrition
Microbes and host genetics
Fecal microbial transplantation (FMT)
Concluding remarks
REFERENCES
Information & Authors
Information
Published In
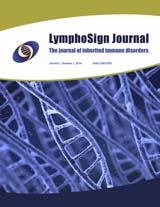
History
Authors
Metrics & Citations
Metrics
Other Metrics
Citations
Cite As
Export Citations
If you have the appropriate software installed, you can download article citation data to the citation manager of your choice. Simply select your manager software from the list below and click Download.
There are no citations for this item
View Options
View options
Login options
Check if you access through your login credentials or your institution to get full access on this article.