Mutations in tetratricopeptide repeat domain 7A (TTC7A) are associated with combined immunodeficiency with dendriform lung ossification but no intestinal atresia
Abstract
Formats available
You can view the full content in the following formats:
REFERENCES
Information & Authors
Information
Published In
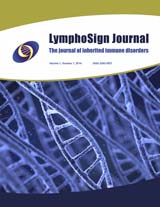
History
Authors
Metrics & Citations
Metrics
Other Metrics
Citations
Cite As
Export Citations
If you have the appropriate software installed, you can download article citation data to the citation manager of your choice. Simply select your manager software from the list below and click Download.
Cited by
View Options
Get Access
Login options
Check if you access through your login credentials or your institution to get full access on this article.