COVID-19 post-vaccination recommendations for primary immunodeficiency
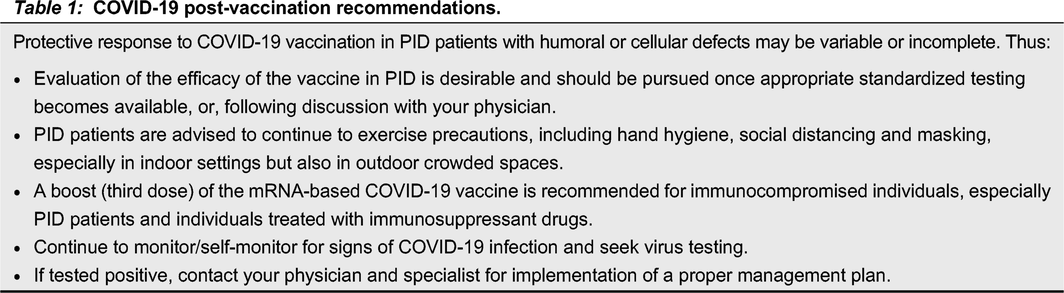
Booster dose of the COVID-19 vaccine
Antibody testing after COVID-19 vaccination
SARS-CoV-2 antibody testing for PID patients
Interpretation of SARS-CoV-2 antibody testing results
Spike protein antibody result: positive
Spike protein antibody result: Negative
Nucleocapsid protein antibody result: positive
Nucleocapsid protein antibody result: negative
REFERENCES
Information & Authors
Information
Published In
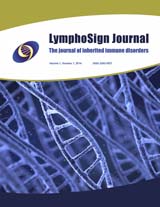
History
Copyright
Authors
Metrics & Citations
Metrics
Other Metrics
Citations
Cite As
Export Citations
If you have the appropriate software installed, you can download article citation data to the citation manager of your choice. Simply select your manager software from the list below and click Download.
Cited by
View Options
View options
Login options
Check if you access through your login credentials or your institution to get full access on this article.