Novel mutation in PIK3CD affecting the Ras-binding domain
Abstract
Introduction
Methods
Patients
Lymphocyte proliferation
Western blotting
Whole exome sequencing and variant calling
Sanger sequencing
Results
Patient 1

Patient 2
Genetics
Cell signaling

Discussion

REFERENCES
Information & Authors
Information
Published In
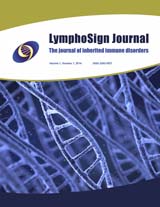
History
Copyright
Authors
Metrics & Citations
Metrics
Other Metrics
Citations
Cite As
Export Citations
If you have the appropriate software installed, you can download article citation data to the citation manager of your choice. Simply select your manager software from the list below and click Download.
There are no citations for this item
View Options
View options
Get Access
Login options
Check if you access through your login credentials or your institution to get full access on this article.